ABSTRACT
Many devices and solutions for remote ECG monitoring have been proposed in the literature. These solutions typically have a large marginal cost per added sensor and are not seamlessly integrated with other smart home solutions. Here we propose an ECG remote monitoring system that is dedicated to non- technical users in need of long term health monitoring in residential environments and is integrated in a broader Internet-of-Things (IoT) infrastructure.
Our prototype consists of a complete vertical solution with a series of advantages with respect to the state of the art, considering both prototypes with integrated front end and prototypes realized with off-the-shelf components: i) ECG prototype sensors with record-low energy per effective number of quantized levels, ii) an architecture providing low marginal cost per added sensor/user, iii) the possibility of seamless integration with other smart home systems through a single internet-of-things infrastructure.
SYSTEM OVERVIEW
The architecture of our IoT platform is illustrated in Fig.1. A detailed description of the platform and of its main components can be found in and. Here, we shall very briefly illustrate the main aspects, with special regard to sensor nodes. The platform has three main parts: the sensor and actuator networks, the IoT server and the user inter faces for visualization and management.
ECG SENSOR DESIGN AND IMPLEMENTATION
The wearable ECG sensor consists of a battery-powered chest belt enabling the measurement and streaming transmission of electrocardiogram signal during daily routines. The belt has two dry plastic electrodes and the electronic printed circuit board (Fig.3).
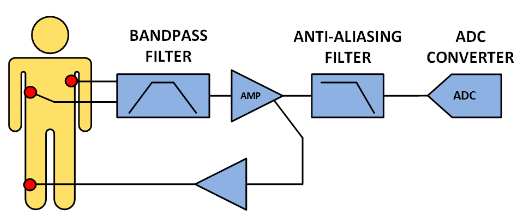
Fig.4 Typical ECG AFE
An instrumentation amplifier with high common-mode rejection ratio (CMRR) is used in the AFE to reduce the common-mode interference. Most systems also typically use a third electrode and a “driven right leg circuit” (DRL) to suppress the interference signal before it can saturate the input amplifier and to further improve the CMRR.
A typical single-channel ECG AFE with DRL circuit is shown in Fig.4. The right leg circuit amplifies the input common mode voltage and feeds it back into the patient’s body, in order to suppress the amplitude of the common-mode interference at the amplifier input.
Since the cutoff frequencies of the input filter depend on the electrode impedances, the values indicated in the figure are indicative.
Our proposed ECG sensor is designed with the aim of minimizing power consumption for a given signal quality, and non-recurrent (design) costs, and this is best obtained with a general purpose ADC chip. The cited ADC is preceded by a passive analog frontend and followed by an MCU, which in turn includes the ZigBee radio transceiver (Freescale MC13224). A power management block completes the system. The main circuit blocks and the analog frontend schematic are shown in Fig.5.
EXPERIMENTAL RESULTS
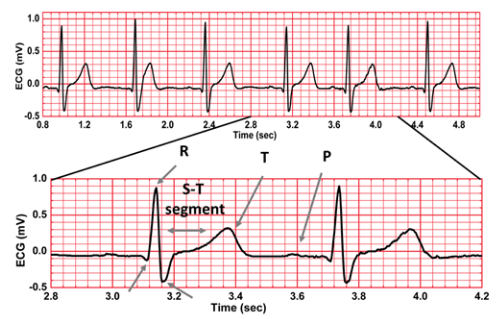
Fig.7 ECG signal waveform
Fig.7 shows the raw measurement data from the ECG sensors recorded from a healthy 35-year old man. As illustrated in Fig.7, the quality of the wireless ECG signal is very good and allows to clearly identify the QRS complex, the P and T waves an d the ST segment.
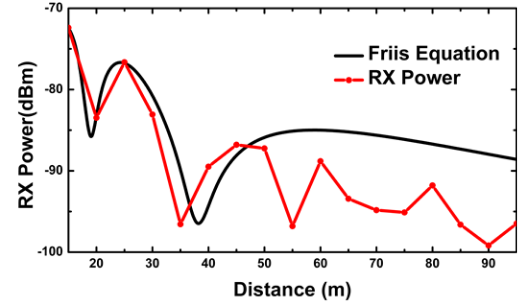
Fig.9 Comparison between measured RX power and Friis equation including ground reflection (considering nominal system parameters and a asphalt relative permittivity of 18)
The outdoor open space range measurement has been performed in an empty parking area, with a low probability of multipath reflect ions from buildings. Both the node and the gateway have been mounted on a plastic pole at a height of 1.5 m from the ground. The average power at the receiver as a function of distance is shown in Fig.9. The effect of ground reflection is clearly visible.
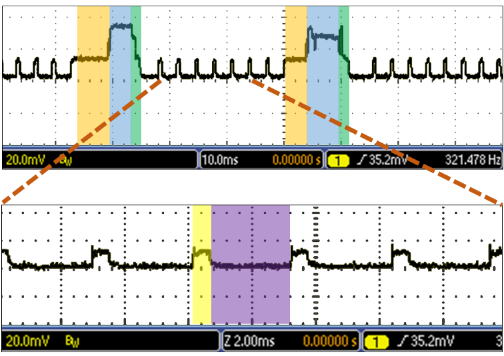
Fig.11 Power consumption during data transmission and acknowledgement
To estimate the suitability of the ECG belt as a long-term wearable sensor, we have evaluated energy consumption. Current draw from the power supply varies during active, transmitting and sleep mode of the device; therefore we have performed the measurement with an oscilloscope.
During the test, the ECG board is powered by a tabletop DC power supply with 3V. The current consumption is measured with an oscilloscope probe over a 1Ω resistor in series with the power supply. The plot in Fig.11 shows the current consumption during data transmission, ECG sampling and MC13224 sleep. Six intervals can be identified during the transmission of a packet without ACK and two during ECG sampling. For each interval, power consumption and duration are measured.
COMPARISON WITH RELATED WORKS
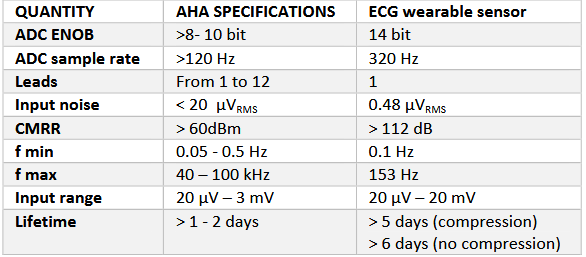
Table 1: Performance Summary of Our ECG Wearable Sensor
The proposed ECG sensor is designed to meet the AHA specifications, as summarized in Table 1. In order to make a comparison with related works on ECG boards, we have selected the prototypes presented in the literature that satisfy the following set of requirements:
- Battery-powered, long term operation (>=24 hours) and wearable;
- Good quality signal (CMRR> = 60 dBm, at least 10 bits, sampling frequency of at least 100Hz);
- Complete sensors (AFE,ADC,MCU, Power Management, Radio or storage unit).
CONCLUSION AND FUTURE WORK
We have proposed a wireless wearable ECG monitoring system embedded in an IoT platform that integrates heterogeneous nodes and applications, has a long battery life, and provides a high-quality ECG signal. The system allows monitoring multiple patients on a relatively large indoor area (home, building, nursing home, etc.).
Our ECG sensor exhibits the record-low EEQNL figure of merit (energy per effective number of quantized levels) of all solutions with both discrete and integrated front ends available in the literature. As we have shown, this result is obtained through a careful set of choices at the level of components, circuit solutions, and algorithms.
We would like to stress the fact that a dedicated front-end chip is not enough to achieve an advantage in terms of overall sensor performance. The latter depends on the optimization of the whole sensor, that is more practically done using off-the-shelf components. Indeed, our proposed ECG sensor, based on a high performance ADC and a microprocessor-radio combo chip, provides much better performance, in terms of power consumption and noise, than many proposed systems based on a purposely designed front end chip.
Another remarkable feature of our system is a very low marginal cost per added sensor, since our architecture enables a single low-cost gateway to manage multiple sensors. Future work will focus on monitoring additional health-related parameters using a broader combination of transducers, sensors, and correlation techniques, and on improving system reliability and robustness to patient movement and connectivity losses.
Source: Wineyard University
Authors: E.Spanò | S.Di Pascoli | G.Iannaccone
>> 200+ IoT Led Projects for Final Year Students
>> More Wireless Sensor Networks Projects Abstract for Final Year Students
>> More Wireless Embedded Projects for Engineering Students
>> More Wireless Energy Projects for Final Year Students