ABSTRACT
This honors thesis presents the design, measurement, and analysis of an openwheeled formula race car suspension system. This race car is the second iteration of Syracuse University’s Citrus Racing student team competition vehicle. The race car’s suspension system features several designs that enable geometric adjustability to impact the vehicle’s dynamic performance.
The purpose of this research is to find an analytic approach to verifying the correlation between suspension design tunings and their effect on vehicle handling and road holding capacity. This was done by analyzing measured data obtained from a system of damper-mounted travel sensors as the vehicle drives through numerous realistic competition scenarios.
MEASUREMENT SET-UP & DATA COLLECTION METHODS
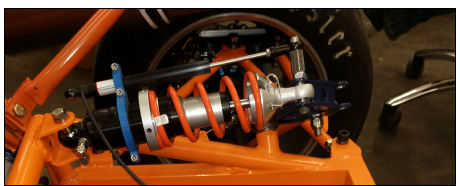
Figure 8: AiM Potentiometer Mounted to the Rear Shock.
The primary measurement sensors used in this thesis are linear potentiometers. They are specifically designed to measure the compression and extension of suspension dampers. The specific sensors used have a diameter of 9.5 mm and measurable stroke length of 75 mm. These linear potentiometers are mounted along the length of the dampers with one end attached to the bolt connecting the damper to the rocker, while the other end is secured to the shock cylinder using a 3D printed, PLA clamp (see Figure 8).
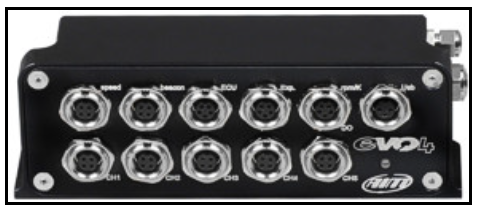
Figure 9: AiM Evo4 Data Logger.
The equipment used for sorting and storing the data is the AiM Evo4 Data Logger (see Figure 9). This on board logger also has a built-in three-axial accelerometer, which collects acceleration data based on the car’s behavior. The Evo4 is bolted to a laminate panel that is clamped to a frame member on the front of the car, oriented in such a way that the accelerometer can accurately collect data. The data logger is connected to the shock pots using 0.5 m 712- 719 patch cords. The Evo4 connects to a laptop using a USB cable.
RESULTS
The results of the measured data analysis will be presented starting with the neutral set-up data. Two alternative test set-ups will then be discussed, one with the greatest achievable anti-squat and anti-dive and one with the highest achievable roll center with the given tuning capabilities. Each set-up had a particular amount of anti- and a particular roll center height set into the system, as prescribed by the designers.
These parameters were hypothesized to affect lateral and longitudinal accelerative capabilities. This correlation will be explored in the Discussion section. The results include plots of peak longitudinal acceleration, peak lateral acceleration, and peak speed. These plots can be found in the appendix. The results also report the effect of this geometry on load transfer using two plots of weight transfer versus acceleration for each set-up.
DISCUSSION
In this section, the data for each tunable parameter is split up in order to look at comparisons across multiple set-ups. Anti-dive, anti-squat, and roll center height data are given in tables. All associated plots, from which the tabular data is drawn, can be found in Appendix A. It should also be understood that the data in this section includes values of average acceleration. Although it might seem like differences on the order 0.01 g of acceleration are insignificant, the cars within the same price range might only see a 0.01 or 0.02 g spread in cornering ability on the skid pad.
CONCLUSION
The most significant conclusion to be made from this experiment is that the basic physics and dynamics on which the original engineering design choices were verified using measured sensor data. In fact, these assumptions are so prominent that the experimental data resoundingly reflected all such trends. These trends included those of the inboard braking stiffness, inboard throttle stiffness, and cornering sensitivity. Overall, it was clear from the comparison between the driver’s notes and the data collected, that some of the fine detail gets lost between the tires and the driver’s notes.
Additionally, it can be concluded from the data that anti-squat geometry has little effect on the amount of throttle acceleration this vehicle can withstand. However, if a car with more torque at the wheels were tested, perhaps one more prone to wheel spin, the results may be different. This is because the race car engine does not have enough accelerative power or torque to make the tires spin. Wheel spin is effectively a car exceeding its capacity to translate power from the drivetrain into motion.
From the tests results of the anti-dive geometry, it was found that the amount of anti-dive tuned into the suspension affected the amount of braking acceleration that the car could hold. Furthermore, it was found that this effect was a negative correlation of braking acceleration with an increased percentage anti-dive. It seems that beyond a certain point, anti-dive is detrimental to the performance of the vehicle. Finally, it was determined that a low roll center height provided the highest level of performance from the vehicle and improvements in drivability. However, there were some unclear trends with the other two set-ups. Because these results are inconsistent, it is encouraged that this area be explored more in the future.
Source: Syracuse University
Authors: Joshua Beckerman